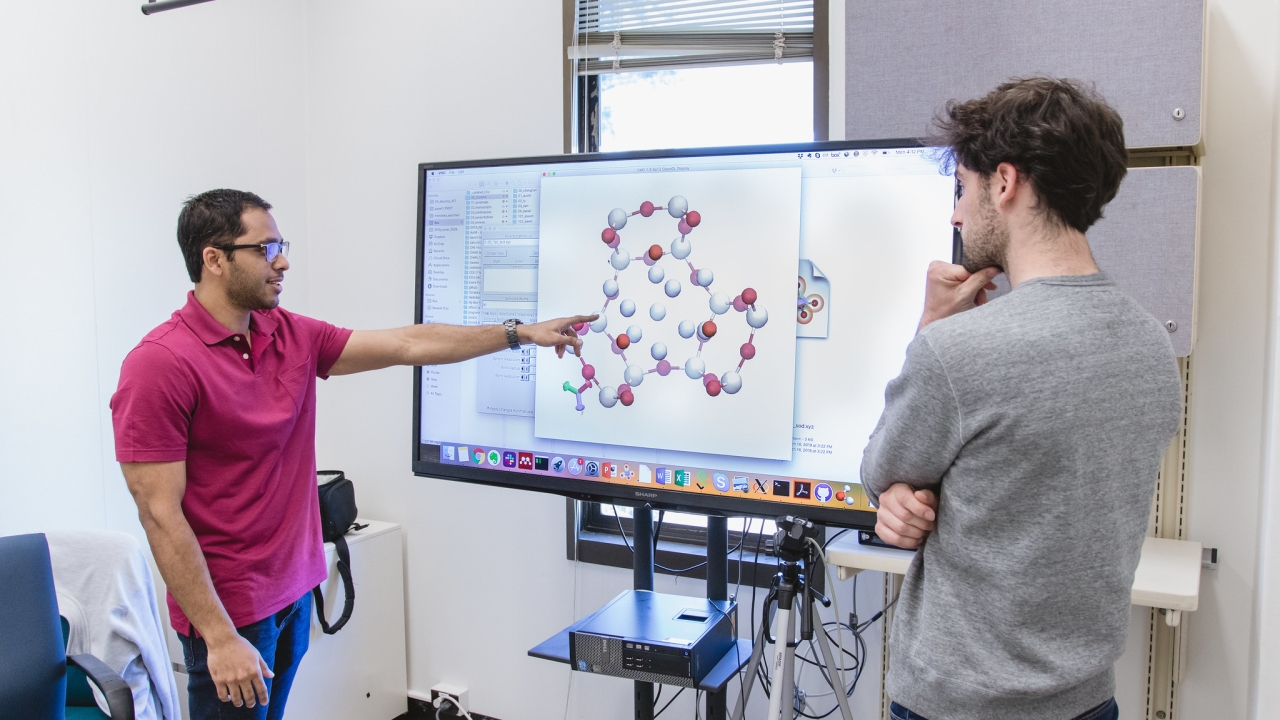
Tackling Climate Change with Chemical Engineering
How Ambarish Kulkarni and his team are using catalyst science to research sustainable climate solutions
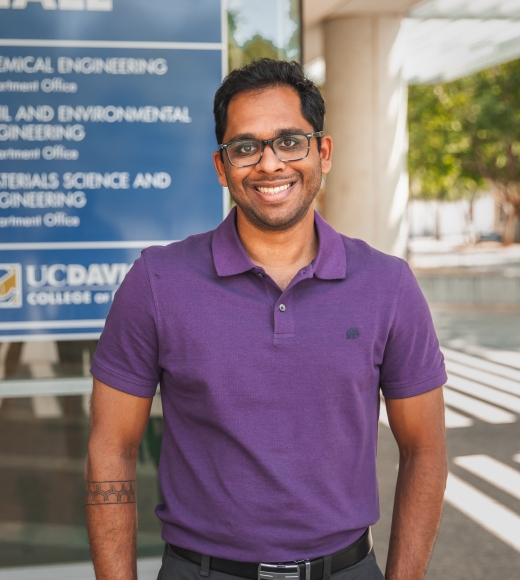
This year's summer was abnormally hot, and now there's talk of an El Niño winter. A recent NASA study reveals that this is no surprise — the ever-rising global emissions of carbon dioxide, or CO2, most of which arise from using fossil fuels, will continue to cause more frequent and more serious climate change-related phenomena.
"There's a big push from the U.S. and other governments to transition our fossil fuel-based economies to something renewable, something more sustainable," says Ambarish Kulkarni, an associate professor of chemical engineering at the University of California, Davis.
Using catalysis and adsorption science, Kulkarni and his team of chemical engineering researchers, with funding from the National Science Foundation, or NSF, the Department of Energy and others, are setting out to help accelerate this clean technology transition, specifically by designing specialized catalysts for fuel cells and adsorbents (typically a solid material that fuses with the molecules of a substance, in this case fuel cells) for carbon capture.
The Backbone of Catalysis
"A catalyst is a material that speeds up a chemical reaction," Kulkarni said. "However, to be practical, the catalyst needs to be sufficiently cheap to produce and the overall processes must be environmentally friendly. The lack of cheaper materials makes it particularly challenging to scale existing technologies."
As an example, Kulkarni describes his experience with the Toyota Mirai. "It's a hydrogen-powered car. Although the overall technology is quite complex, the operating principle is very straightforward. Basically, you take hydrogen fuel and combine it with oxygen to produce water, and in that conversion, you produce energy — which provides the 'driving force' for the car."
However, current fuel cell technologies, like the one in the Mirai, rely on expensive platinum-based catalysts. Thus, a major goal in Kulkarni's work, supported by his NSF CAREER award, is to accelerate the discovery of cheaper fuel cell catalysts that may eventually result in affordable zero-emission cars, which can be more widely adopted.
Reducing Carbon Emissions
In addition to reducing the emissions from the mobility sector, there is a lot to be done in how energy is produced and consumed. For example, the CO2 produced by burning fossil fuels from coal and natural gas power plants has contributed significantly to various environmental challenges, including the uptick in severe and extreme weather events that are all too common today.
While alternative technologies for large-scale electricity generation are being explored, the chemical and manufacturing sectors will continue using fossil fuels to provide industrial heat over the next decade or so.
"It is likely that some form of carbon capture will be necessary," Kulkarni said. "Given that the switch to renewable energy sources will be a slow process, it is possible that in addition to reducing our carbon emissions, we will need novel 'net-negative' technologies to remove CO2 that has already been emitted directly from the atmosphere."
Investigating these novel technologies, called direct air capture, or DAC, has received significant support from the federal government. In this domain, Kulkarni and his team of collaborators are studying a class of materials called zeolites. These adsorbents act as sponges that selectively bind CO2 from a complex mixture of gases. The challenge, however, is water.
"When you burn fuel, you will always have water as a by-product — it is unavoidable. You can remove it by drying, but it's really expensive and hard, and when there's water present, the CO2 adsorption capacity of zeolites crashes," he said.
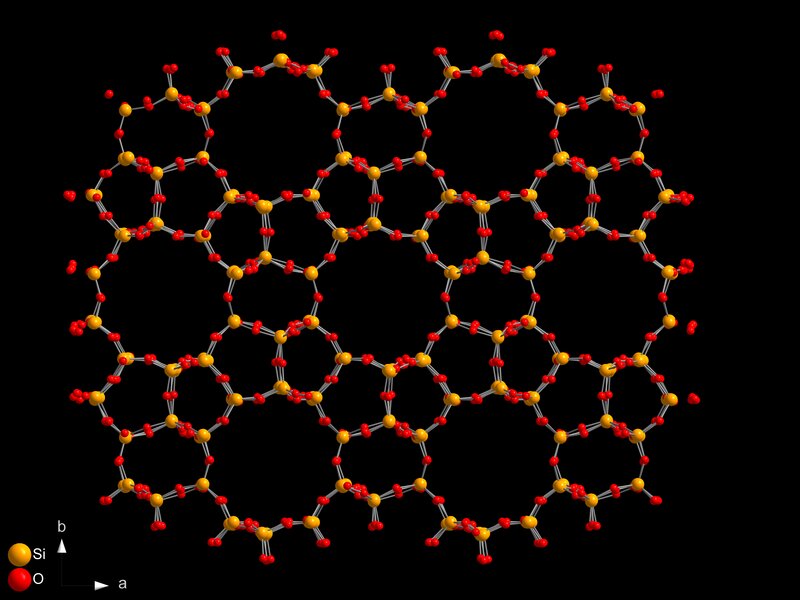
However, in studying different zeolites under wet and dry conditions, Kulkarni and his collaborator Alexander Katz, a professor of chemical and biomolecular engineering at the University of California, Berkeley, discovered that one type of zeolite material shows an increase in CO2 uptakes in the presence of small quantities of water.
This surprising phenomenon is due to the formation of new binding sites for CO2 that are only formed when a small amount of water is also present, explained Kulkarni (a research paper on this topic is currently being prepared for publication).
Although this technology is not yet a commercially viable process, his team is now studying whether this phenomenon is more general and can be used to design even better adsorbents for CO2 capture and ideally a material capable of directly capturing CO2 from the air and converting it into a usable energy source.
"It's a big technological advance if you're able to capture CO2 in the presence of water," Kulkarni explained.
Materials by Design
Kulkarni's pursuit is aided by the application of multiscale atomistic modeling, which is being done in collaboration with his UC Davis colleagues: chemical engineering associate professors Coleman Kronawitter and Ron Runnebaum. This strategy uses a series of complex computer simulations based on quantum chemistry to computationally design better materials and is used for two purposes.
"Typically, we use computer simulations to try to explain interesting experimental observations," Kulkarni said. "Then, we try to use this knowledge to screen large libraries of materials rapidly."
The hope is that such accelerated design of materials will lead to faster discoveries when compared to traditional trial-and-error approaches. Along with good catalytic and adsorption properties, Kulkarni's team aims to design materials that will lend toward better performance and stability, cheaper costs and more environmentally sustainable technologies.
Making predictions is one part of the process; making and testing the materials in the lab is the other, which is no small task. To make the same material each time, a precise recipe would need to be followed.
"The main challenge within my community, I would argue, is that we don't quite understand, at an atomistic level, how functional materials are actually formed in the lab," Kulkarni said.
Kulkarni now hopes to use machine learning-based tools to develop predictive models that will allow theorists to provide synthesis recipes to guide the experimental realization, and not just computational design, of materials in the laboratory.
Due to his research into clean energy opportunities, Kulkarni has been invited to apply for the NSF 2024 Trailblazer Engineering Impact Award program (selections will likely be made in the spring), which supports selected engineers in research projects that have the potential to "innovatively and creatively address major society changes and make major contributions toward solving significant research problems." Kulkarni thinks his research and the chemical engineering community can contribute significantly towards this grand challenge.
"It is an exciting time to be a chemical engineer," Kulkarni says. "With all the hype around artificial intelligence and the world being at the cusp of a green-energy revolution, we need all the smart people working on this."